#50: Plasma Catalysis, CCUS for Industries, Compressed Air Energy Storage
Plasma Catalysis for CO2 conversion; ConsenCUS - industrial clusters with CCUS in Europe; Climate impact on rail infra in the UK; Compressed Air Energy Storage in China
This is the 50th issue of Global Climate News. The top 5 most viewed posts cover
Reducing emissions from road freight transport, shipping, aviation, chemicals and petrochemicals
Tidal Stream Energy and Finland’s Sand-based thermal storage
Thank you for reading! Let me know if you have any thoughts or feedback in comments or email soumya@telborg.com
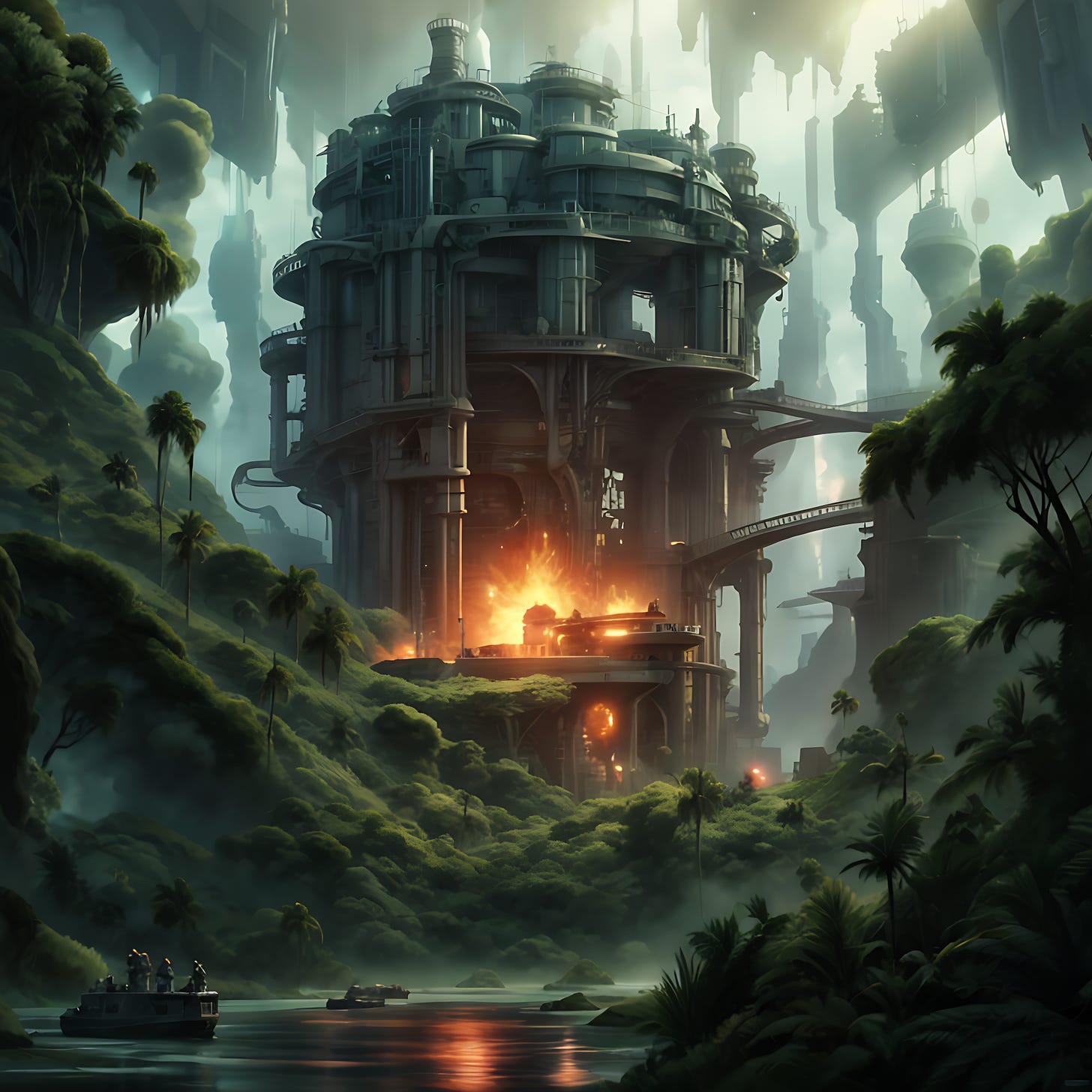
Plasma Catalysis for making hydrogen and methane
Plasma is a state of matter (besides the 3 well-known states - solid, liquid, gas), so high energy that atoms in it separate into ions and electrons. It’s a
gas which is at least partially ionized and contains various types of particles, viz. electrons, atoms, ions and molecules. Plasma as a whole is electrically quasi-neutral.
Electrically ‘quasi-neutral’ because the ions and electrons taken as a whole give plasma which has no net charge, but within the plasma many particles (ions and electrons) carry charges.
Carrying out reactions in the plasma state is beneficial as the particles are already in the high-energy state needed for many reactions, such as high-temperature electrolysis and pyrolysis. In traditional high-temperature catalysis, the reaction intermediates (reactive species) are formed when adsorbed on the surface of the catalyst, whereas in plasma catalysis these can form directly in the plasma state due to very high energy, before interaction with the catalyst. Less energy is needed then to convert the intermediates to final products.
But the presence of lots of reactive particles means many reaction products can be formed, so catalysts are used to direct the reaction towards specific products.
Consider ammonia synthesis, a process carried out globally at industrial scales (150 million metric tons produced in 2022) using the highly energy-intensive Haber-Bosch process which requires temperatures of 700 Kelvin and a pressure of 100-200 atm.
it has been estimated that this (Haber-Bosch) process produces more than 300 million tons of CO2 annually and consumes up to 2% of the world's energy. Src
Using plasma catalysis instead of Haber-Bosch, ammonia can be made at room temperature and pressure. Another benefit it that plasma catalysis is efficient even at small scale, unlike the Haber-Bosch process which is efficient only with large-scale reactors.
Plasma catalysis has been used commercially for making ozone since the 2000s and is being researched extensively for ammonia synthesis, methane reformation for producing hydrogen, water treatment, making nanomaterials, disinfection and more. Many steps in semiconductor manufacturing also make uses of plasmas.
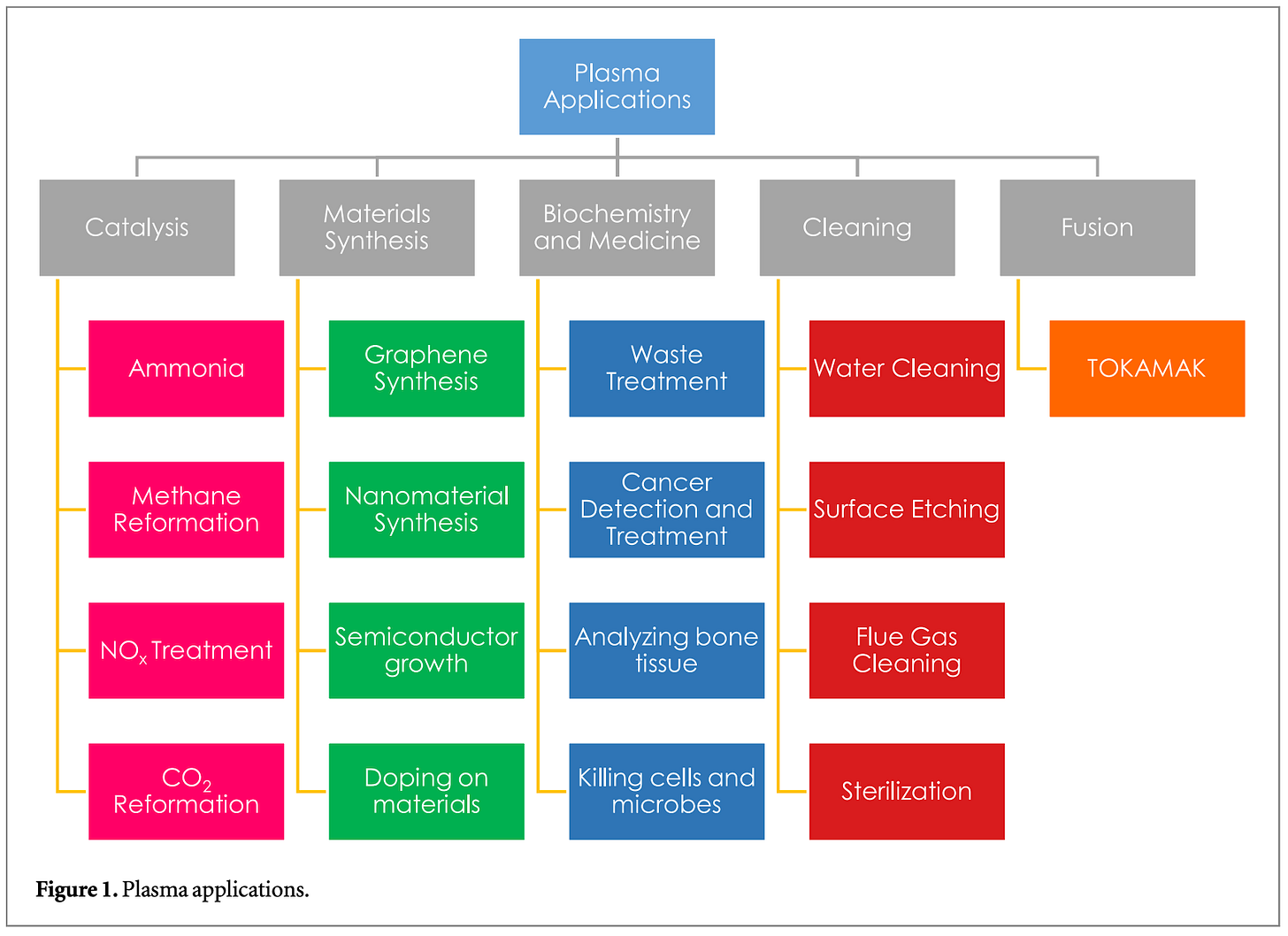
In the lab, the plasma state is achieved by subjecting gases, such as helium, to high-voltage electric currents.
The type of plasma discharges generated in the laboratory are typically produced by driving an electrical current through a gas. In these discharges, the energy from the electric field is first accumulated by the electrons and then it is transferred from electrons to heavy particles by collisions. During a collision between a heavy particle and an electron, the latter lose only a small portion of its energy, due to their small mass(lighter). This explains why initially the temperature of the electron is higher that the heavy components. Later, if time and energy are enough, subsequent collisions (Joule heating) can equilibrate their temperatures.
When the ions and electrons have the same high temperature, you get a thermal plasma with a temperature between 3000-5000 Kelvin. These are generated using high-voltage currents at very high pressures, and are used in fusion reactors.
At room temperature and pressure, passing high-voltage current through a gas gives a ‘cold plasma’ - the bulk of the gas is at room temperature while the electrons are at temperature of 10^5 Kelvin.
There are many types of plasmas including dielectric barrier discharge, microwave plasma, gliding arc plasmas and more, depending on the frequency of radiation used to produce them and the configuration of the reactor.
Dielectric Barrier Discharge (DBD) reactors have a dielectric material in between the electrodes, and run at atmospheric pressure and room temperature producing non-thermal (cold) plasma. Although gliding arc and microwave plasmas have better energy efficiency for CO2 conversion, the latter operate at much higher temperatures upto 3000 Kelvin, and cannot be used with catalysts.
France-based Energo is using DBD plasmas to produce hydrogen from 2 paths - one using water, the other from ammonia. Earlier, Energo built a prototype to convert CO2 into methane. CO2 is a very stable molecule and it takes a lot of energy to convert into other molecules, such as methane, in reactions. The typical reaction (Sabatier reaction) for converting CO2 to methane is done at 3MPa (mega pascal) pressure and 300-400℃ temperature. Using plasma, this can be done at room temperature and pressure. Most of the energy in a plasma is held by the electrons, and electrons in a high-energy state cause otherwise stable molecules such as CO2 to dissociate and form new molecules.
Other benefits of plasma catalysis include fast start and stop times, which allow them to be used when needed rather than in continuous operation. The CAPEX is low and no rare materials are needed. To scale up plasma catalysis for commercial use, more reactors can be added in parallel. The process is efficient both at small and large scale which means you can use it in small setups, in combination with local solar installations to provide energy.
The technology has not scaled commercially yet as better catalysts are needed to achieve high efficiency and selectivity i.e. directing the reaction towards a specific desired product.
Offshore Energy | Energo | Plasma-based CO2 conversion (video) | Plasma Catalysis: A Brief Tutorial
ConsenCUS - setting up Industrial clusters with CCUS
The ConsenCUS project aims to setup net-zero industrial clusters in North-West and South-East Europe. At the project locations, carbon dioxide will be captured at the emissions source (such as oil refining, cement production and magnesite production facilities) using a biodegradable sorbent, potassium hydroxide. The captured CO2 will be converted into useful chemicals like potassium formate, using renewable energy to provide the electricity needed. The project is also looking into the transport infrastructure, such as pipelines, needed for transporting CO2, and analysing different rock types to determine which formations can be used for underground CO2 storage. Interesting findings from ConsenCUS Policy Paper 1, published in 2023:
Current mature CO2 capture technologies use solvents such as amines, that are volatile, toxic and require energy to regenerate the solvent i.e. to remove the captured CO2 so they can be used again
Water-based electrochemical CO2 capture methods are limited by their high electricity consumption
Carbon capture technologies are assessed on their CO2 capture rate and purity of the CO2 stream released, but have to make “trade-offs between the performance, energy consumption, and resource needs”.
The energy consumption required for high capture rates (“energy penalty”) is well-known but other trade-offs must also be considered: for example, adding certain chemicals to the solvents used for capture can increase CO2 absorption efficiency at the expense of solvent regeneration efficiency, and using electricity means that the rate of regeneration will be limited by the maximum power available at industrial sites. This shows that the applicability of CO2 capture is dependent on a number of factors, many of which involve trade-offs and increase the complexity of decision-making.
Countries like the UK have minimum capture efficiency requirements for commercial CCUS projects, which limits the choice of technologies.
To assess the net emissions of a CCUS pathways one must consider origin of the carbon, emissions of the energy sourceand the final storage or product. A pathway contributes to lowering emissions only if the carbon comes from an atmospheric or biological source and renewable energy is used to power the process. If the captured carbon is used in concrete, carbon is locked up for longer periods than if used for synthetic fuels.
ConsenCUS has a demonstration unit at a Portland cement facility in Aalborg (Denmark) that is using electrochemical carbon capture technology. Electrochemical methods operate at lower temperatures and use much less energy (~50% lower) than more common carbon capture methods.
The method involves “washing” the CO2 flue gas with liquid potassium hydroxide. This binds the CO2 to the liquid. The CO2-containing liquid is then fed into an “electrochemical trap/regenerator” where power is applied to the liquid. Thus, CO2 is cleaved from the liquid, and the two elements can then be separated. CO2 is subsequently sent into a new electrochemical cell, where CO2 is converted into formic acid.
Compressed Air Energy Storage (CAES)
CAES is based on a relatively simple idea
In times of excess electricity on the grid (for instance due to the high power delivery at times when demand is low), a compressed air energy storage plant can compress air and store the compressed air in a cavern underground. At times when demand is high, the stored air can be released and the energy can be recuperated. Src
The air released is used to drive a turbine generator, generating electricity when required. The earliest CAES projects are the Huntorf plant in Germany (commissioned 1978) and the McIntosh plant in Alabama, USA (commissioned 1991). Both use underground salt caverns to store the compressed air.
China has now connected the largest CAES plant, a 300MW facility, to the grid. The plant can provide upto 6 hours of energy backup to nearly 200,000-300,000 at peak consumption, and also uses as salt cavern as storage.
Based on CAES technology, Italy-based Energy Dome has built a CO2 battery. Excess electricity is used to compress CO2 into a liquid (at high pressures) and when energy is needed the CO2 is allowed to vaporise and drive a turbine generator. America’s Westinghouse is also working on a CO2-based long duration storage.
(Between salt caverns for storage, reservoirs for hydrogen and CO2 storage, and geothermal wells, sub-surface real estate is really picking up.)
Top Stories
France, Germany and Italy have allocated a combined €2.5bn funding for projects in mining, processing and recycling critical minerals including copper, lithium, battery metals and rare earths. The fund is expected to launch this summer. Euronews
Flooding due to extraordinarily heavy rains in the UK is damaging rail lines, while earlier, extreme heat caused track lines to buckle. Network Rail, which operates Britain’s rail network, has allocated £2.8bn over the next 5 years for “activities and technology to help it cope with the impact of climate change”.
The new investment will also go into building or rebuilding more than 600,000 metres of drains and recruiting more than 400 extra drainage engineers, who will boost the amount of maintenance done of drains so they can handle heavier rainfall.
More than 20,000 cuttings and embankments have been targeted for repair work, with more than 300 miles being strengthened.
The investment marks a major increase from the £1bn that Network Rail had initially earmarked for spending on climate change during the five-year period to April 2029, and is nearly six times the £500m in the investment plan that ran from 2019 to 2024.
Metal-organic frameworks are used as sensors for detection of toxic gases, as they can absorb gases and their pore size can be designed for selectively capturing specific gases. But once saturated, MOFs no longer work as effective sensors. In new research, MOFs were combined with conductive polymers (organic polymers that conduct electricity), resulting in a composite that was both sensitive to gases, and could be used for detection after multiple cycles. MIT News | Research in Advanced Materials
The US will introduce tariffs on the import of bifacial solar modules into the country. These were previously excluded from anti-dumping tariffs, and accounted for almost all recent solar panel imports into the country. Mercom | White House Press Release
China has launched an anti-dumping investigation into the imports of polyoxymethylene copolymer from EU, the US, Taiwan and Japan. The chemical is a synthetic polymer used in mechanical gears, vehicle parts, medicine, food packaging, sports equipment and across many industries. ET Energy
You can find all previous posts of this newsletter here.
I’m the maker of SummaryWithAI.com. You can find me on Twitter and LinkedIn.